Astrophysicists unveil the unexpected symmetry of cosmic explosions
Although the magnificent explosion of colliding neutron stars is visible across most of the Universe, the resulting fireball is much too small to study in detail. But now astronomers from the Cosmic Dawn Center has found a way to measure the shape of the explosion. The surprising result provides new insight into fundamental physics relating to the formation of heavy elements and the birth a black holes. The study has just been published in the scientific journal Nature.
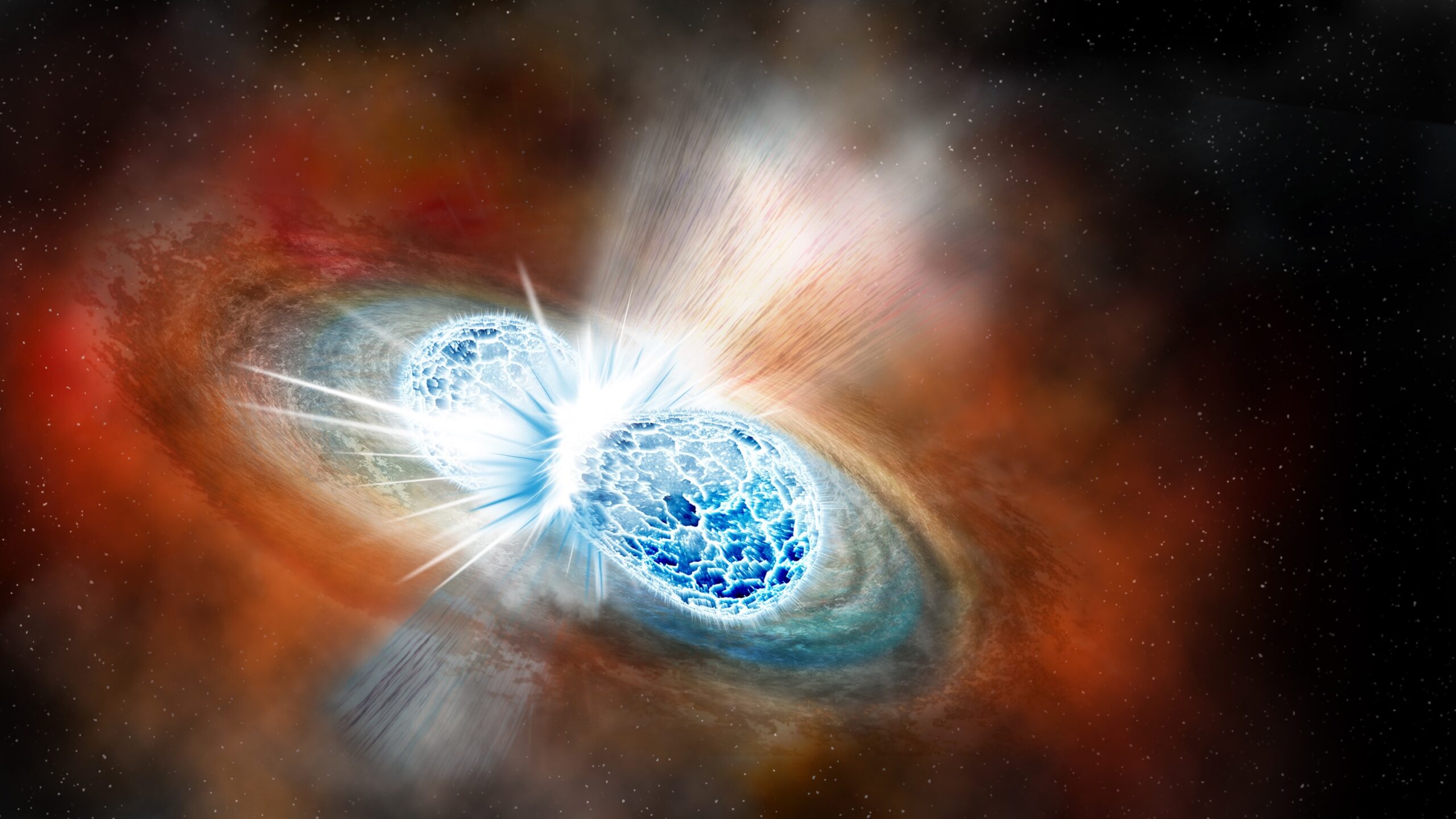
Artist’s impression of two neutron stars which, after having spiraled inwards for billions of years, end their lives in an immense explosion known as a kilonova. Most heavy elements such as the gold on your finger is thought to be created in this process. Illustration: Robin Dienel (Carnegie Institution for Science).
When a massive star exhausts its fuel, it ends its life not with a whimper but with a bang.
While the star’s outer layers are blown out at tremendous speeds in the surrounding space, the core collapses to an extremely compact neutron star (or even a black hole).
A neutron star weighs the same as our Sun or more, but is squeezed into a ball only 10 kilometers across.
If two such compact objects orbit each other, they will eventually spiral in and collide. The result is yet another bright explosion, known as a kilonova, followed by a collapse of the innermost parts to a black hole.
Kilonovae: a new field of study
Although the existence of kilonovae was predicted in 1998, the first observation was only confirmed in 2013. Four years later, the new gravitational wave observatories LIGO in the US and Virgo in Europe, detected gravitational waves from kilonovae, characteristic of massive objects merging. It was realized that, contrary to what was thought at the time, kilonovae are the Universes’ main factory for producing heavy elements such a gold, silver, platinum, uranium, and plutonium.
Theoretical and observational studies have brought us closer to an understanding of this enigmatic phenomenon. But since all observed kilonovae, to date, have been detected at vast distances — the closest one more than 100 million lightyears away — we are unable to see the details of the explosion.
Because the progenitor of the kilonova are two stars orbiting in a plane, we might expect the explosion cloud to be inherently asymmetric. This hypothesis is supported by computer simulations and other theoretical calculations.
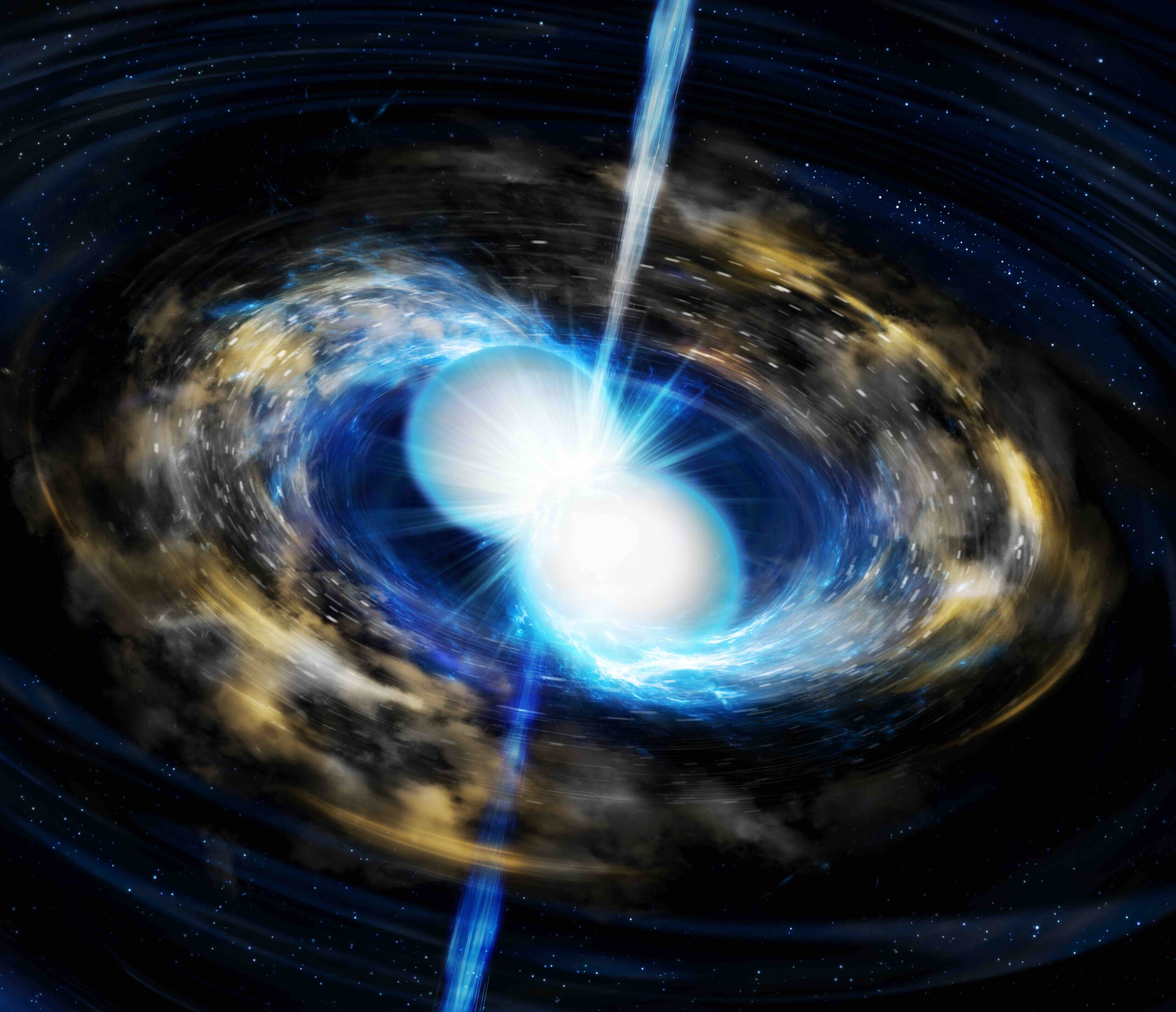
Most models predict that centrifugal forces should eject neutron star material in the plane of their orbit, shortly before the rest collapses to a black hole. Illustration: Tohoku University.
Spherical explosion
But now astrophysicists from the Cosmic Dawn Center in Copenhagen challenge this belief:
Analyzing the spectrum — that is, the colors of the light dispersed according to its wavelength — of an already well-studied kilonova dubbed AT2017gfo they find not one, but two signatures that reveal the shape of the erupting fireball.
And the result was surprising — even to themselves!
The first method was to measure the velocity of the expanding fireball in two different directions; along the line of sight, and perpendicular to this, respectively.
The study was led by PhD student Albert Sneppen who explains: “Along the line of sight, this is quite a standard procedure in astronomy, as the velocity induces a Doppler shift which is seen in the spectrum. In the direction perpendicular to our sightline it’s a bit more tricky, because telescopes can’t resolve the explosion. But by combining the different channels of information — the gravitational waves it emitted, the radio waves at later times, the distance to the host galaxy, and the brightness of the explosion —we could infer how its area increased, and hence how fast it is expanding.”
The astronomers found the two velocities to be the same to within a few percent. And if a cloud expands with the same velocity in different directions, it must be spherical.
The Doppler effect
The Doppler shift is a well-known phenomenon from sound: The pitch of, say, an ambulance driving toward you is higher than a stationary ambulance, because the wavelength of the sound is “compressed”.
A similar effect exists for light: If a luminous object is traveling toward you, its light will acquire a shorter wavelength and hence a change in color. Comparing the observed color with what it is “supposed” to be allows you to deduce the exact velocity.
For instance, a green photon emitted by an object traveling at 20% the speed of light toward you will be shifted to a blue photon. On the other hand, if the object is receding from you at this speed, you will observed a red photon.
For this reason, we also say that the light is blueshifted or redshifted, respectively.
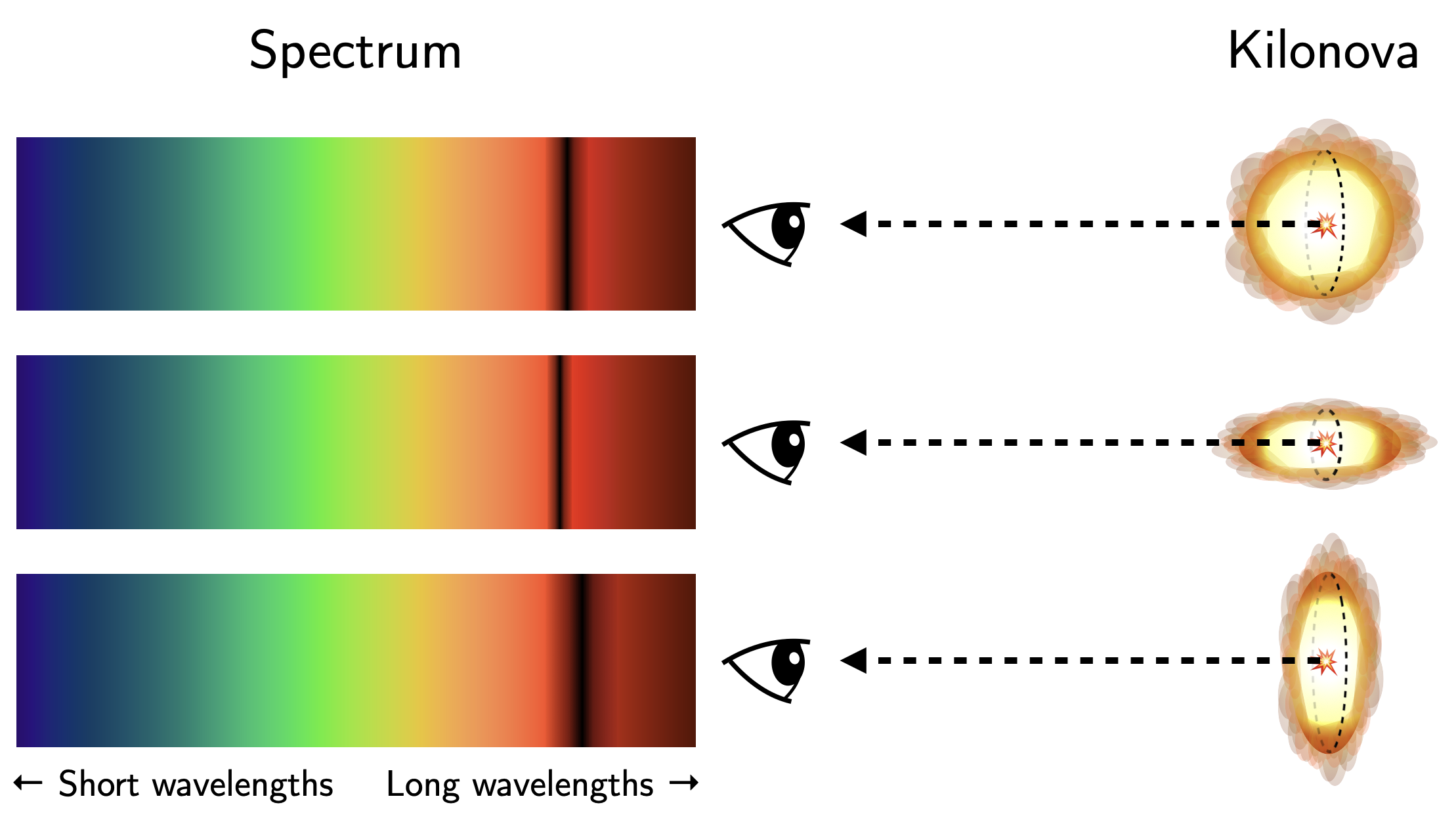
Elements in the expanding cloud absorb some of the light emitted from the center of the explosion. For strontium, which is abundant in the cloud, this absorption happens around wavelengths of 0.8 micron. But if the cloud is not spherical, the absorption may be shifted to shorter or longer wavelengths, depending on the shape. In this figure the effect is exaggerated, and in reality the dark absorption line is farther out to the right, in the infrared region. Illustration: Peter Laursen (Cosmic Dawn Center).
Independent methods yield the same result
To verify the unexpected result, Albert Sneppen and his colleagues took a closer look at a specific feature in the spectrum caused by strontium, an element which was previously used to prevent X-ray emission from color TVs, and today is commonly used to make red fireworks. This element is mixed within the gaseous, expanding cloud, absorbing part of the light emitted from the center of the explosion.
“It turns out that the exact wavelengths where gas in the expanding cloud absorbs light from behind reveals the shape of the cloud,” Albert Sneppen explains. “If the cloud is elongated like a rugby ball, the absorption is shifted a little toward shorter wavelengths. On the other hand if it’s flattened like a M&M candy (those without peanuts), the absorption extends longer wavelengths.”
But the astronomers found the absorption to shifted neither direction, indicating again that the explosion is symmetric.
Fundamental physics at play
Which physical process could lead the the observed symmetry is yet unknown, but the researchers do have a few possible mechanisms on the drawing board:
“We don’t know yet what’s going on, except that the usual assumption of energy released by radioactive decay is not enough,” says Darach Watson, associate professor at the Cosmic Dawn Center and second-author on the study. “Perhaps a neutron star collapsing to a black hole could cause a ‘magnetic bomb’ when magnetic fields are cut and suddenly reconnect outside the black hole. Perhaps fast-spinning neutron stars can prolong the collapse for a split-second, resulting in a hypermassive neutron star that may create an immensely powerful wind. And perhaps the extreme densities can affect the way the ultra-light neutrino particles escape. All these mechanisms are worth exploring.”
The result not only provides new insight into the fundamental physics of these immense explosions, the genesis of heavy elements, and the birth of black holes. It also holds the key to learning about another interesting phenomenon, namely the expansion of the Universe.
“Current methods to measure the cosmic expansion rate disagree, yielding different values. We think the fact that kilonovae are near-spherical in shape will allow us to use how their brightness evolves with time to make completely independent estimates of the expansion rate, resolving this major cosmological question,” Darach Watson elaborates.
The study has just been published in the scientific journal Nature.
Mere information
Contact
Publication
- Sneppen et al. (2023): Spherical symmetry in the kilonova AT2017gfo/GW170817
Danish version
To read this news story in Danish, click here.
Tags: Kilonovae